Gaussian Integral with Complex Offset
Theorem:
![]() |
(D.12) |
Proof:
When
, we have the previously proved case. For arbitrary
and real number
, let
denote the closed rectangular contour
, depicted in Fig.D.1.
Clearly,
is analytic inside the region bounded
by
. By Cauchy's theorem [42],
the line integral of
along
is zero, i.e.,
![]() |
(D.13) |
This line integral breaks into the following four pieces:
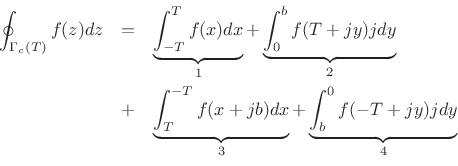
where
and
are real variables. In the limit as
,
the first piece approaches
, as previously proved.
Pieces
and
contribute zero in the limit, since
as
. Since the total contour integral is
zero by Cauchy's theorem, we conclude that piece 3 is the negative of
piece 1, i.e., in the limit as
,
![]() |
(D.14) |
Making the change of variable

![]() |
(D.15) |
as desired.
Next Section:
Fourier Transform of Complex Gaussian
Previous Section:
Integral of a Complex Gaussian